Sepsis, a life-threatening syndrome induced by infection, has been a leading cause of hospitalization and death in U.S. healthcare settings and accounts for more hospital admissions and spending than any other condition.1,2 Early and effective diagnosis and treatment of sepsis, along with prevention, have been a patient-safety focus to improve outcomes and reduce mortality. Unfortunately, sepsis remains a significant challenge within the medical community due to its elusive nature and rapid progression.2 The heterogeneous presentation of sepsis often impedes efforts for early diagnosis and management.3
As a harbinger of the future, precision medicine (or personalized medicine) first came to light in 1999 when specific genetic markers and therapeutics were identified. In 2015, it became a national initiative under the Obama administration and was identified as one of the research priorities by the Surviving Sepsis Campaign in 2023. Precision medicine is an innovative approach tailoring disease prevention and treatment to a patient’s genes, environments, and lifestyles.4 As one size does not fit all, the goal of precision medicine is to target the right treatments to the right patients at the right time. This article aims to review current diagnostic methods and discuss emerging techniques in precision medicine in sepsis diagnosis.
Current challenges in sepsis diagnosis
The causal pathogen, infection location, associated organ dysfunction, antimicrobial resistance profiles, and host factors collectively encompass the heterogeneity of sepsis presentation and progression. When the infection source remains unclear, imaging studies, including X-rays, CT scans, MRIs, or ultrasounds, can be instrumental.5 These factors and the time needed for culture and serology tests contribute to a range of outcomes, necessitating various types and intensities of treatment.6 This heterogeneity has likely added to the failure of multiple pharmacologic and supportive care interventions in critically ill patients.7 Additionally, there are limits to current diagnostic methods and definitions (Table 1) making sepsis a challenge to diagnose while potentially exposing some patients to unnecessary antibiotics and care pathways. According to the U.S. Centers for Disease Control (CDC), approximately 30% of the antibiotics administered in the U.S. are unnecessary or suboptimal, causing 2.8 million antibiotic-resistant infections annually and more than 35,000 deaths.8
Precision medicine technologies in sepsis diagnosis
Patients with sepsis exhibit a spectrum of dysfunctional responses ranging from an exaggerated hyperinflammatory state to immunoparalysis. Precision medicine examines the patient at the molecular level to provide pinpoint accuracy in diagnosis and treatment. Several emerging technologies have shown the potential to tailor treatments to individual patient characteristics. Multiple “-omics” technologies, sometimes called multi-omics (e.g., epigenomics, transcriptomics, proteomics, metabolomics), combine these methods for diagnosis and management.
Genomics
Genomic technologies study the totality of a person’s DNA. Though inflammatory biomarkers are elevated in both sepsis and sterile inflammation, variances in gene expression signatures could differentiate between the two entities and improve understanding of disease susceptibility.
An initial study of 11 gene sets showed a mean area under the curve (AUC) of 0.87 to distinguish systemic inflammatory response syndrome (SIRS) due to trauma from SIRS due to sepsis.11 Of the genes tested, FAM20A+OLAH show good performance with an AUC of 0.9906 and could be used to triage patients rapidly who have suspected severe inflammation, while the CMTM5/CETP/PLA2G7/MIA/MPP3 gene panel could differentiate SIRS from sepsis with an AUC of 0.9758.12 Interestingly, urine can also be used to discern gene expression during early sepsis from inflammatory SIRS, instead of using blood.13
The genomics of vasomotor dysregulation in septic shock has been examined. The Vasopressin and Septic Shock Trial identified two single nucleotide polymorphisms (SNPs) with the homozygous genotype of rs28418396 associated with severe adverse events with vasopressor use and the homozygous genotype of ADRB2 rs1042717 associated with a higher risk of mortality. However, in vitro, the administration of low-dose steroids eliminated this genotypic effect.14,15 Glucocorticoids impact the vascular tone and reduce pro-inflammatory gene transcription. However, SNPs affecting receptor function can have negative impacts on septic patients, though in-vitro use of methylprednisolone overcame this effect.16 However, there have been no prospective, in-vivo, validation trials to assess therapeutic regimen adjustments based on SNP information.
Epigenomics
Epigenomics is a rapidly expanding field exploring heritable changes in gene expression due to the environment, allostatic load, and other external factors that do not alter DNA sequencing and could explain the variability in gene expression during sepsis.
Investigations into various microRNAs, an epigenetic marker, indicate their expression is linked to sepsis-associated encephalopathy, acute lung injury, acute kidney injury, and endothelial leakage, suggesting that these could be potential targets for therapeutic development.17 Exosome mimic therapy, which packages beneficial microRNAs to deliver them to targeted cells in septic patients similar to its use in cancer treatment, is gaining interest.18
Transcriptomics
Transcriptomics analyzes and monitors RNA transcription to gain insights into regulatory mechanisms, disease pathways, and therapeutic targets. This field has helped identify several different sepsis phenotypes and endotypes. Moreover, it is established that gene expression endotypes observed on day one differ from those on day three among half of adult septic patients.19
The U.K. Genomic Advances in Sepsis study identified two sepsis response signature (SRS) phenotypes. SRS1 individuals had suppressed immune system response (higher T-cell exhaustion), and have a higher 14-day mortality risk, and SRS2 are those with normal immune system response.20 However, in the Vasopressin vs Norepinephrine as Initial Therapy in Septic Shock trial, mortality risk increased significantly for SRS2 phenotypes when given steroids compared to SRS1.21
The MARS (Molecular Diagnosis and Risk Stratification of Sepsis) Consortium revealed four different molecular endotypes, with MARS1 (BPGM, TAP2) having the worst outcome/more immunosuppressed, MARS2 (GADD45A, PCGF5) and MARS4 (IFIT5, GLTSCR2) exhibiting an exaggerated inflammatory state and MARS3 (AHNAK, PDCD10) correlated with better outcomes and appropriate adaptive immune function.22
Two recent studies are advancing rapid sepsis diagnosis. The first study categorized suspected sepsis patients into five endotypes based on unique gene expression within two hours of their arrival in the emergency department, showing varying outcomes.23 The second study introduced a rapid testing system with a one-hour turnaround time, using four host immune markers to diagnose sepsis. It was validated against Sepsis-2 and Sepsis-3 screening tools on ICU patients, demonstrating high predictive value for sepsis diagnosis, although its usefulness for patients with lymphopenia or neutropenia is limited.24
Proteomics
By elucidating protein structures, modifications, and interactions, proteomic analyses offer insights into disease mechanisms and potential therapeutic targets.
Through the use of this technology, acute respiratory distress syndrome patients have been classified into two phenotypes: hyperinflammatory (elevated IL-6, IL-8, and sTNFR-1 levels, protein C deficiency, metabolic alkalosis, increased prevalence of shock, thrombocytopenia, and higher vasopressor use); and hypoinflammatory (lower IL-6 and IL-8 levels, normal protein C levels, higher survival rate), which directs the care pathway patients receive regarding positive end-expiratory pressure, the fluid management strategy, and simvastatin use.25 Also, the reclassification of the Prospective Recombinant Human Activated Protein C Worldwide Evaluation in Severe Sepsis—Shock study population revealed that the hyperinflammatory acute respiratory distress syndrome group had a lower mortality rate with activated protein C administration compared to the hypoinflammatory group.7
As some septic patients have a severe hyperinflammatory response, representing a macrophage-activation-like syndrome as indicated by high ferritin levels, the pilot study Personalized Randomized Trial of Validation and Restoration of Immune Dysfunction in severE infections and Sepsis (PROVIDE) showed some benefit from anakinra administration to improve Sequential Organ Failure Assessment (SOFA) score at seven days of drug use, though with no benefit in mortality.26 The ImmunoSep, or Personalised Immunotherapy in Sepsis, trial is a double-blind, multinational, randomized, controlled trial based on the PROVIDE pilot study to investigate the use of biomarkers (ferritin and HLA-DR) and adjunctive immunotherapy (recombinant interferon-gamma and anakinra) to improve outcomes in fulminant hyperinflammation or immunoparalysis sepsis subgroups. This trial may demonstrate a better response with a large study population and a longer treatment window.27
Metabolomics
Sepsis affects several metabolic and biochemical pathways, and investigating the activation, suppression, and dysregulation of these cycles has the potential to enhance diagnosis, risk stratification, and treatment. Cellular metabolism of amino acids, lipids, and carbohydrates is altered in sepsis, leading to increased oxidative stress. This pattern distinguishes septic patients from those with sterile SIRS and healthy subjects.28 Metabolomic profiling has also shown promise in sepsis diagnosis, with studies demonstrating that different metabolic fingerprints can be associated with early septic shock diagnosis compared to other types of shock.29 Elevated levels of 3-hydroxybutyrate, alpha-1-acid glycoprotein, and glycine, along with a decrease in the concentration of citrate and histidine, are potential markers that could facilitate the identification of early-stage sepsis phenotypes.30
Microbiome analysis
Changes in the microbiome have been associated with sepsis, suggesting that microbiome analysis could be a valuable tool in sepsis diagnosis. Diet, medications, and environment also impact the health of an individual’s microbiome. In septic patients, gut dysbiosis leads to a dysregulated inflammatory response, impaired immunomodulation, organ dysfunction, and altered metabolism due to a rapid and persistent decrease in short-chain fatty acid concentration.31 Enterococcus species’ predominant dysbiosis was positively correlated with increased gene expression related to cell chemotaxis and inflammatory damage.32 Potential therapeutics targeting microbial function modification may involve select small-molecule inhibition of specific enzymes, harvesting microbial bacteriocins as novel antibiotics, or administering microbial product analogs such as receptor agonists to mimic the beneficial effects of microbiota.33
Cytomics
Cytomics studies the immune cell responses to sepsis triggers. Current areas of research include low-density neutrophils, monocyte human leukocyte antigen-DR isotype, and toxic granulocyte production in neutrophils. Low-density neutrophils are present in chronic inflammatory and autoimmune states but are also seen in sepsis. They contribute to a weakened immune response (reduced chemotaxis, phagocytic capacity, and T cell proliferation) and proinflammatory state (increased reactive oxygen species).34 Reduced or deficient monocyte human leukocyte antigen-DR isotype expression impairs antigen presentation for immune signaling and reduces tumor necrosis factor-alpha (TNF α) production.35 Intensive-care-unit populations with severe deficiencies had higher mortality risk, longer length of stay, and higher risk for secondary infections.26
Machine learning
Machine learning algorithms can integrate large volumes of data and identify patterns that might not be apparent to clinicians, thereby aiding in early sepsis diagnosis. Dr. Mihir Patel explains more about machine learning and digital tools in his article, Digital Tools: The New Frontline Against Sepsis.
Point-of-care testing for biomarkers
Several biomarkers have been used to identify and differentiate patients with sepsis.
Guidelines caution about the risks of false positives and negatives when using procalcitonin (PCT), emphasizing the need for careful interpretation of results in the appropriate clinical context as noted in Table 2.36,37 Patients on dialysis without an active infection can have a PCT level >0.5.38 Three separate meta-analyses of PCT for diagnosing sepsis showed a sensitivity range of 77% to 85% and a specificity range of 75% to 83%.39
C-reactive protein (CRP) is another biomarker used to assess for sepsis. Levels rise quickly following inflammatory insult, though they are not affected by immunosuppression, chronic kidney disease, end-stage renal disease, or cirrhosis and cannot reliably distinguish between sepsis and SIRS, with a sensitivity of 78% to 80% and specificity of 60% to 61%.40 A meta-analysis of monocyte distribution width revealed higher sensitivity but lower specificity compared to PCT, with similar sensitivity and specificity compared to CRP.41 Point-of-care neutrophil CD64 testing in emergency department patients shows promise for ruling in bacterial infections, with a positive predictive value of 1, sensitivity of 0.27, specificity of 1, and negative predictive value of 0.64.42
Cell-free DNA techniques have enhanced the care of septic patients by enabling rapid diagnosis of sepsis-causing pathogens along with their antibiotic-resistance profiles compared to standard blood culture methods. A recent proof-of-concept study showed a reduced time to sensitivity of 12 hours to allow for earlier targeted therapy.43
Although the concept of precision medicine is often used to classify patients by molecular means, physiologic measurements remain valuable to developing this approach by aiding in patient therapeutic selection criteria due to advances in monitoring, signal processing, and imaging techniques.44 Vital signs or temperature trajectory can assist in stratifying the risk of septic patients and identifying those who would benefit from balanced fluids.45,46
Future perspectives and conclusion
The 2023 White House Office of Science and Technology Policy Report outlined goals to advance precision medicine, including health monitoring, multi-omics, cell therapies, artificial-intelligence–driven drug development, and microbial genome sequencing.47 Many published studies are limited by research design, focusing on selective biomarkers or pathways and small sample sizes, which restrict their broad application. Additionally, clinical variables and measurable “-omics” must offer actionable information and predictable responses so as to customize care and enhance patient outcomes.44 The ethical issue of equipoise arises when using biomarker credentials to randomize patients who may be genetically disadvantaged and unable to benefit from the experimental therapy.44 There are several prospective studies of various patient characteristics and “omics” which are evaluating the feasibility of real-time identification that could influence decision making and assessment of targeted therapies in clinical settings.27,44,48-51 However, there is a need for integration with a multi-omics approach to capture the complex relationship between different biological systems to have a transformative impact on the understanding and management of sepsis.
Ethical challenges and precautions in applying precision medicine in sepsis should be considered. If the use of predictive biomarkers enhances patient care and outcomes, there are ethical, legal, and social implications that involve integrating these diagnostic and therapeutic options responsibly, equitably, and effectively.52 It is recommended that decisions to apply a precision-medicine approach, like any other test or treatment option, should be guided by the best interests of the patient, scientific evidence, and ethical standards.53 These considerations, including risks, limitations, and benefits, are inclusive of the physician’s ethical responsibility to practice high-value care.53 Additionally, more inclusive research is needed to ensure the generalizability of specific markers and thresholds for diagnosis and initiation of therapeutics.
In summary, precision medicine accelerates the process of obtaining crucial information about hosts, genes, and microbes from days to just hours, enhancing patient care by customizing treatment. This strategy targets reduced antimicrobial resistance, patient morbidity, and healthcare costs.
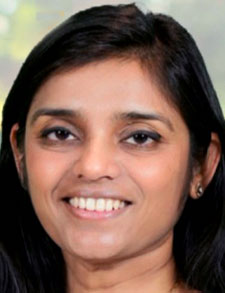
Dr. Iyer
Dr. Iyer is a hospitalist in internal medicine and an associate clinical professor at UCI Health in Orange, Calif.
References
- Gale B, Hall KK. Sepsis recognition. In: Hall KK, Shoemaker-Hunt S, et al. Making Healthcare Safer III: A Critical Analysis of Existing and Emerging Patient Safety Practices [Internet]. Rockville, MD: Agency for Healthcare Research and Quality (US); 2020;(3)1-22. https://www.ncbi.nlm.nih.gov/books/NBK555517/
- Singer M, Deutschman CS, et al. The third international consensus definitions for sepsis and septic shock (Sepsis-3). JAMA. 2016;315(8):801-10.
- Rhodes A, Evans LE, et al. Surviving sepsis campaign: international guidelines for management of sepsis and septic shock: 2016. Intensive Care Med. 2017;43(3):304-77.
- S. Food & Drug Administration. Precision medicine. FDA website. https://www.fda.gov/medical-devices/in-vitro-diagnostics/precision-medicine. Updated September 27, 2018. Accessed July 31, 2024.
- Vincent JL, Sakr Y, et al. Prevalence and outcomes of infection among patients in intensive care units in 2017. JAMA. 2020;323(15):1478-87.
- Biebelberg B, Rhee C, et al. Heterogeneity of sepsis presentations and mortality rates. Ann Intern Med. 2024;177(7):985-7.
- Sinha P, Meyer NJ, et al. Biological phenotyping in sepsis and acute respiratory distress syndrome. Annu Rev Med. 2023;74:457-71.
- S. Centers for Disease Control and Prevention. Core elements of hospital antibiotic stewardship programs. CDC website. https://www.cdc.gov/antibiotic-use/hcp/core-elements/hospital.html . Published March 14, 2024. Accessed July 31, 2024.
- Levy MM, Fink MP, et al. 2001 SCCM/ESICM/ACCP/ATS/SIS International Sepsis Definitions Conference. Crit Care Med. 2003;31(4):1250-6.
- Rodenberg H, Glasser T, et al. Sepsis-2.5: Resolving conflicts between payers and providers. Crit Care Explor. 2023;5(9):e0970. doi:10.1097/CCE.0000000000000970
- Sweeney TE, Shidham A, et al. A comprehensive time-course-based multicohort analysis of sepsis and sterile inflammation reveals a robust diagnostic gene set. Sci Transl Med. 2015;7(287):287ra71. doi:10.1126/scitranslmed.aaa5993
- Szakmany T, Fitzgerald E, et al. The ‘analysis of gene expression and biomarkers for point-of-care decision support in sepsis’ study; temporal clinical parameter analysis and validation of early diagnostic biomarker signatures for severe inflammation and sepsis-SIRS discrimination. Front Immunol. 2024;14:1308530.
- Bandyopadhyay S, Loftus TJ, et al. Early differentiation between sepsis and sterile inflammation via urinary gene signatures of metabolic dysregulation. Shock. 2022;58(1):20-7.
- Anantasit N, Boyd JH, et al. Serious adverse events associated with vasopressin and norepinephrine infusion in septic shock. Crit Care Med. 2014;42(8):1812-20.
- Nakada TA, Russell JA, et al. Beta2-adrenergic receptor gene polymorphism is associated with mortality in septic shock. Am J Respir Crit Care Med. 2010;181(2):143-9.
- Baker AC, Chew VW, et al. Single nucleotide polymorphisms and type of steroid impact the functional response of the human glucocorticoid receptor. J Surg Res. 2013;180(1):27-34.
- Isac S, Isac T, et al. The omics complexity in sepsis: the limits of the personalized medicine approach. J Pers Med. 2024;14(3):225.
- Paskeh MDA, Entezari M, et al. Emerging role of exosomes in cancer progression and tumor microenvironment remodeling. J Hematol Oncol. 2022;15(1):83.
- Stevens J, Tezel O, et al. Biological basis of critical illness subclasses: from the bedside to the bench and back again. Crit Care. 2024;28(1):186.
- Davenport EE, Burnham KL, et al. Genomic landscape of the individual host response and outcomes in sepsis: a prospective cohort study. Lancet Respir Med. 2016;4(4):259-71.
- Antcliffe DB, Burnham KL, et al. Transcriptomic signatures in sepsis and a differential response to steroids. From the VANISH randomized trial. Am J Respir Crit Care Med. 2019;199(8):980-6.
- Scicluna BP, van Vught LA, et al. Classification of patients with sepsis according to blood genomic endotype: a prospective cohort study. Lancet Respir Med. 2017;5(10):816-26.
- Baghela A, Pena OM, et al. Predicting sepsis severity at first clinical presentation: The role of endotypes and mechanistic signatures. EBioMedicine. 2022;75:103776.
- Balk R, Esper AM, et al. Validation of SeptiCyte RAPID to discriminate sepsis from non-infectious systemic inflammation. J Clin Med. 2024;13(5):1194.
- Calfee CS, Delucchi KL, et al. Acute respiratory distress syndrome subphenotypes and differential response to simvastatin: secondary analysis of a randomised controlled trial. Lancet Respir Med. 2018;6(9):691-8.
- Leventogiannis K, Kyriazopoulou E, et al. Toward personalized immunotherapy in sepsis: The PROVIDE randomized clinical trial. Cell Rep Med. 2022;3(11):100817.
- Kotsaki A, Pickkers P, et al. ImmunoSep (Personalised Immunotherapy in Sepsis) international double-blind, double-dummy, placebo-controlled randomised clinical trial: study protocol. BMJ Open. 2022;12(12):e067251. doi: 10.1136/bmjopen-2022-067251.
- Hussain H, Vutipongsatorn K, et al. Patient stratification in sepsis: using metabolomics to detect clinical phenotypes, sub-phenotypes and therapeutic response. Metabolites. 2022;12(5):376.
- Mickiewicz B, Tam P, et al. Integration of metabolic and inflammatory mediator profiles as a potential prognostic approach for septic shock in the intensive care unit. Crit Care. 2015;19(1):11.
- Jaurila H, Koivukangas V, et al. 1H NMR based metabolomics in human sepsis and healthy serum. Metabolites. 2020;10(2):70.
- Haak BW, Wiersinga WJ. The role of the gut microbiota in sepsis. Lancet Gastroenterol Hepatol. 2017;2(2):135-43.
- Sun S, Wang D, et al. Altered intestinal microbiome and metabolome correspond to the clinical outcome of sepsis. Crit Care. 2023;27(1):127.
- Kitsios GD, Morowitz MJ, et al. Dysbiosis in the intensive care unit: microbiome science coming to the bedside. J Crit Care. 2017;38:84-91.
- Murao A, Aziz M, Wang P. Neutrophil heterogeneity in sepsis: the role of damage-associated molecular patterns. Shock. 2023;59(2):239-46.
- Lindell RB, Meyer NJ. Interrogating the sepsis host immune response using cytomics. Crit Care. 2023;27(1):93.
- Khilnani GC, Tiwari P, et al. Guidelines for the use of procalcitonin for rational use of antibiotics. Indian J Crit Care Med. 2022;26(Suppl 2):S77-S94.
- Chambliss AB, Patel K, et al. AACC guidance document on the clinical use of procalcitonin. J Appl Lab Med 2023;8:598-634.
- Grace E, Turner RM. Use of procalcitonin in patients with various degrees of chronic kidney disease including renal replacement therapy. Clin Infect Dis. 2014;59(12):1761-7.
- Póvoa P, Coelho L, et al. How to use biomarkers of infection or sepsis at the bedside: guide to clinicians. Intensive Care Med. 2023;49(2):142-53.
- Kim JH. clinical utility of procalcitonin on antibiotic stewardship: a narrative review. Infect Chemother. 2022;54(4):610-20.
- Huang YH, Chen CJ, et al. Comparison of the diagnostic accuracies of monocyte distribution width, procalcitonin, and C-reactive protein for sepsis: a systematic review and meta-analysis. Crit Care Med. 2023;51(5):e106-e114. doi:10.1097/CCM.0000000000005820
- van de Ven NLM, Bongers SH, et al. Point-of-care neutrophil CD64 as a rule in diagnostic test for bacterial infections in the emergency department. BMC Emerg Med. 2023;23(1):28.
- Sonntag M, Elgeti VK, et al. Suppression PCR-based selective enrichment sequencing for pathogen and antimicrobial resistance detection on cell-free DNA in sepsis-a targeted, blood culture-independent approach for rapid pathogen and resistance diagnostics in septic patients. Int J Mol Sci. 2024;25(10):5463.
- Gordon AC, Alipanah-Lechner N, et al. From ICU syndromes to ICU subphenotypes: consensus report and recommendations for developing precision medicine in ICU. Am J Respir Crit Care Med. 2024;210(2):155-66.
- Bhavani SV, Wolfe KS, et al. Temperature trajectory subphenotypes correlate with immune responses in patients with sepsis. Crit Care Med. 2020;48(11):1645-53.
- Bhavani SV, Semler M, et al. Development and validation of novel sepsis subphenotypes using trajectories of vital signs. Intensive Care Med. 2022;48(11):1582-92.
- The White House Office of Science and Technology Policy. Bold goals for U.S. biotechnology and biomanufacturing. The White House website. https://www.whitehouse.gov/wp-content/uploads/2023/03/Bold-Goals-for-U.S.-Biotechnology-and-Biomanufacturing-Harnessing-Research-and-Development-To-Further-Societal-Goals-FINAL.pdf. Published March 2023. Accessed August 1, 2024.
- Bhavani S. Precision resuscitation with crystalloids in sepsis (PRECISE). U.S. National Library of Medicine Clinical Trials registration website. https://www.clinicaltrials.gov/study/NCT06253585. Published February 2, 2024. Updated July 24, 2024. Accessed August 1, 2024.
- RWTH Aachen University. Prospective, randomized trial of personalized medicine with Pentaglobin® after surgical infectious source control in patients with peritonitis (PEPPER). National Library of Medicine clinical trials registration website. https://www.clinicaltrials.gov/study/NCT03334006. Published November 2, 2017. Updated June 21, 2024. Accessed August 1, 2024.
- Koutroulis I. Biomarker-enhanced artificial intelligence based pediatric sepsis screening tool. National Library of Medicine clinical trials registration website. https://www.clinicaltrials.gov/study/NCT05311046. Published March 31, 2022. Accessed August 1, 2024.
- Bhavani S. Novel sepsis sub-phenotypes based on trajectories of vital signs. National Library of Medicine clinical trials registration website. https://www.clinicaltrials.gov/study/NCT05826223. Published April 12, 2023. Updated May 17, 2024. Accessed August 1, 2024.
- Boyce AM, Garibaldi BT. Genomics and high-consequence infectious diseases: a scoping review of emerging science and potential ethical issues. Health Secur. 2019;17(1):62-8.
- Lehmann LS, Snyder et al. Ethical considerations in precision medicine and genetic testing in internal medicine practice: a position paper from the American College of Physicians. Ann Intern Med. 2022;175(9):1322-3.